Posted At: Tem 25, 2023 - 583 Views
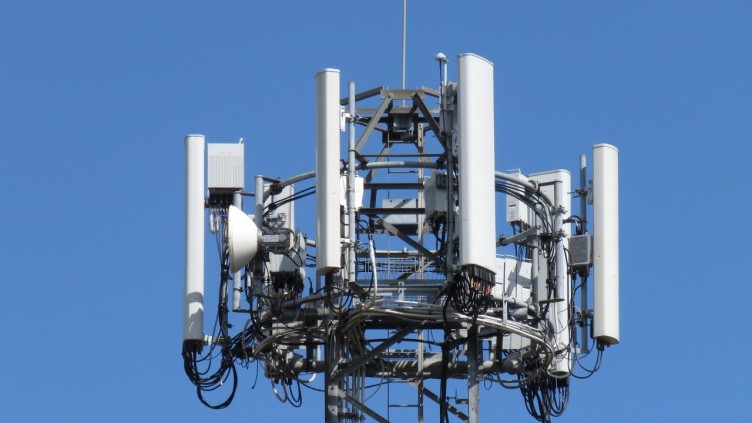
Wireless network systems introduced users to multiple-input multiple-output (MIMO) transmission and reception techniques, superseding the single antenna transmit/receive channel with two, three, or four transmit/receive channels. MIMO allows the systems to achieve higher data throughputs and improve signal quality with minimal additional hardware. Today, the same concepts are being applied to cellular communications, but with more emphasis on increasing the number of channels, such that the term massive MIMO is becoming mainstream.
But What is Massive MIMO?
Though there are no exact definitions for “massive,” many companies apply the term to Tx/Rx arrays of from 16 to 256 or more channels. As Allen Tran, the VP of Engineering, and Joe Burke, a Principal Engineer, both from Qualcomm, explain, each channel is an active antenna with transmit/receive capabilities. And when configured in an array (4 × 4, 8 × 16, 16 × 16, 32 × 32, for example), control electronics and digital-signal processing can electronically manipulate the phase of the array to adjust the direction of the radiated signal (beamsteering) and provide spatial multiplexing (Fig. 1).
This generic massive-MIMO radio antenna system consists of an active antenna array (far right) that interfaces to multiple radio paths consisting of signal splitters, bandpass filters, transmit/receive switches, and power amplifiers/low-noise amplifiers. A digital baseband modem (far left) incorporates logic that manages the multi-user massive MIMO with beamforming weights. Outbound data streams are converted to analog and transmitted, while received analog signals are digitized sent to the modem. (Courtesy of Qualcomm)
This generic massive-MIMO radio antenna system consists of an active antenna array (far right) that interfaces to multiple radio paths consisting of signal splitters, bandpass filters, transmit/receive switches, and power amplifiers/low-noise amplifiers. A digital baseband modem (far left) incorporates logic that manages the multi-user massive MIMO with beamforming weights. Outbound data streams are converted to analog and transmitted, while received analog signals are digitized sent to the modem. (Courtesy of Qualcomm)
The multiple antenna elements can thus focus their energy into smaller regions of space. That improves the energy efficiency from the increased antenna gain by bundling the transmitted energy. Massive MIMO takes advantage of uncorrelated propagation paths for higher efficiency and high throughput and/or to allow simultaneous access for different users.
But why use it? Systems get improved energy efficiency by bundling the transmitted energy. That also increases the range and reduces inter-cell interference. At higher frequencies (millimeter wave, or mmWave) there’s a concern about higher path loss. Still, the smaller antenna size at the higher frequencies could permit designers to build larger arrays to compensate. As a result, at higher frequencies, the larger number of antenna elements can generate a very narrow beam with a large gain. And at lower frequencies, the large number of antenna elements can generate multiple spatial streams.
However, many challenges arise in order to implement and test a massive-MIMO system. For starters, over-the-air testing (as opposed to standard conducted testing) should be done to ensure the beams are pointing in the right direction. At high frequencies, conducted testing becomes more challenging and expensive due to the high losses associated with the cables and other aspects of system implementation. In addition, given a large number of antenna elements, beamforming antennas that aren’t properly calibrated will generate unwanted emissions in unwanted directions (referred to as beam squint), which is basically jitter of the beam boresight.
Test Issues During Design and Production
In the design phase, conducted testing is done when antenna connectors are still accessible. A vector network analyzer (VNA) measures S-parameters (both transmission and reflection coefficients). When combined with a switch matrix, the combo can be used to measure the S-parameters of antenna arrays up to 288 elements. The S-parameters contain magnitude and phase information that can be applied to measure both near-field and far-field quantities.
Reflection coefficient = S11 = b1/a1 (reflected power at antenna 1/ injected power at antenna 1)
Transmission coefficient = S21 = b2/a1 (transmitted power at antenna 2/injected power at antenna 1)
VNAs provide flexible test solutions, allowing for the choice of either a true multiport vector network analyzer or a switched-matrix solution. Multiport VNAs simultaneously measure all ports to reduce the test duration and perform a complete mutual coupling measurement between one antenna element and its surrounding neighbors.
An additional benefit is that specific tests like “active return loss” (S11, S22, S33, …, S2424) can be measured in parallel with many ports stimulated simultaneously. This method provides deeper insights into an antenna array in the design phase, which affects the real-world operation case in terms of network capacity.
Mutual coupling between the antenna elements results in energy loss and thus a reduction in the maximum range. Tests to determine phase adjustments can help minimize coupling losses. Sometimes, the array’s physical shape may have to take on non-geometric shapes, resulting in energy dissipation in undesired directions. Testing using a VNA and spectrum analyzer will help determine where unwanted signals are being sent and allow system calibration to minimize the lost energy.
An antenna array applying massive MIMO in both the sub-6-GHz and mmWave frequency range will not provide antenna connectors due to added complexity and cost, physical size limitations, and resulting insertion loss. Consequently, over-the-air (OTA) tests are required. OTA tests measuring the three-dimensional antenna pattern can be performed either in near-field or far-field. When performing measurements in the near-field, smaller anechoic chambers can be used, but require an additional near-field to far-field transformation for antenna gain patterns.
OTA Measurements Split Between R&D and Production
OTA measurement parameters can be divided into two general categories: R&D for a more complete investigation of the device-under-test radiated properties, and production for calibration, verification, and functional testing. The main tests during the R&D phase include Gain Patterns, Effective Radiated Power, Receiver Sensitivity, Transceiver and Receiver characterization, and Beam Steering and Beam Tracking. For production testing, the main activities include Antenna/Relative Calibration, Transceiver Calibration, Five-Point Beam Test, and various Functional Tests.
Gain patterns are either 2D from one of the three principal planes (E1, E2, or H-plane) or a complete 3D pattern. For antenna arrays with one or more beams, the 3D gain pattern is more useful. The effective radiated power (ERP) or effective isotropic radiated power (EIRP) is used to measure an active antenna system either as user equipment (UE) or a base station. For UE testing, total radiated power (TRP) is employed instead, where TRP is the weighted integral of the ERP values over a sphere.
Receiver sensitivity is characterized by the parameter effective isotropic sensitivity (EIS) and measures the block-error-rate as a function of the received power equal to the specified receiver Massive MIMO: Design and Test Challenges 5 sensitivity. Each individual transceiver in the active antenna system needs to be verified through an OTA interface.
Precise beam tracking and fast beam acquisition, due to the high path loss and limited range of a mmWave wireless system, is required for mobile users. Although antenna implementations for existing cellular technologies can employ static beam-pattern characterization, mmWave systems will require dynamic beam measurement systems.
Functional tests are the final tests performed on the completely assembled unit in production. These tests can consist of a simple radiated test, a five-point beam test, and an aggregate transceiver functionality test, such as an EVM measurement of all transceivers.
The distance between the antenna under test and the measurement antenna is relevant for OTA transmissions. The very near-field, or reactive field, is the result of electromagnetic coupling at a distance smaller than the first Fresnel zone, with a typical range of only a few centimeters. This field isn’t suitable for OTA measurements since the measurement antenna becomes part of the radiating elements. Measurements in the adjacent radiated near-field depend on magnitude and phase.
Multiple sample measurements on a specific trajectory or surface and some post-processing must be done to convert the data to far-field (near-field to far-field transformation). The Fraunhofer distance, which is the border between the near-field and far-field, is calculated as 2*D2/λ, where D is the aperture size of the antenna array and λ is the wavelength (Fig. 2)
Measurement aspects in the near-field and far-field depend on chamber size. For near-field tests, multiple samples are required along with near-field to far-field post-processing. For far-field testing, only a single sample is needed with no near-field/far-field post-processing.